New science facilities are needed to keep America great
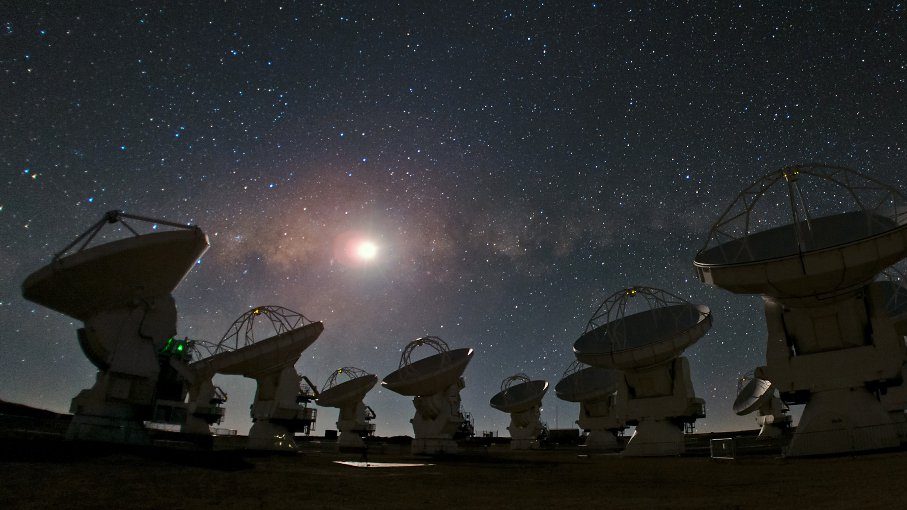
- For decades, the National Science Foundation has been the driving force in the construction of new science facilities across the country, including our greatest solar, radio, optical, and gravitational wave telescopes.
- However, science continues to advance, and here — a quarter of the way into the 21st century — new, superior discovery tools are coming across the board: from China, Europe, Japan, and elsewhere.
- It’s now a critical moment for the United States: will we continue to be leaders in science, including astronomy? Or will we surrender that science leadership to other countries across the world?
Some of the biggest questions in the Universe — some of which have been pondered since the dawn of humanity — are finally within reach here in the 21st century. Is there life out there in the Milky Way beyond our own Solar System? How were the elements that made complex life and biochemistry possible created throughout our Universe’s history? Are there inhabited worlds, perhaps with intelligent civilizations on them, that we can potentially visit and study? Are giant pairs of orbiting supermassive black holes creating a gravitational wave background in our Universe? And, if we build the scientific tools required to answer these questions, what else, perhaps even unexpectedly, could we discover?
Here in 2025, we’re now a quarter of the way through the 21st century. The greatest science facilities of the prior generation — including space telescopes, ground-based optical and radio telescopes, and gravitational wave detectors — have brought us up to the current scientific frontier. We’ve discovered thousands of exoplanets, measured billions of stars in our own galaxy, discovered galaxies and quasars tens of billions of light-years away, detected the existence of a background gravitational wave signal rippling through space, and measured neutron stars and black holes merging from all across the Universe.
So what comes next for a fundamental science like astronomy in the USA: the leader on all of these fronts for the past 75+ years? That all depends on whether we maintain our promise to keep America great. Here’s what that would look like.
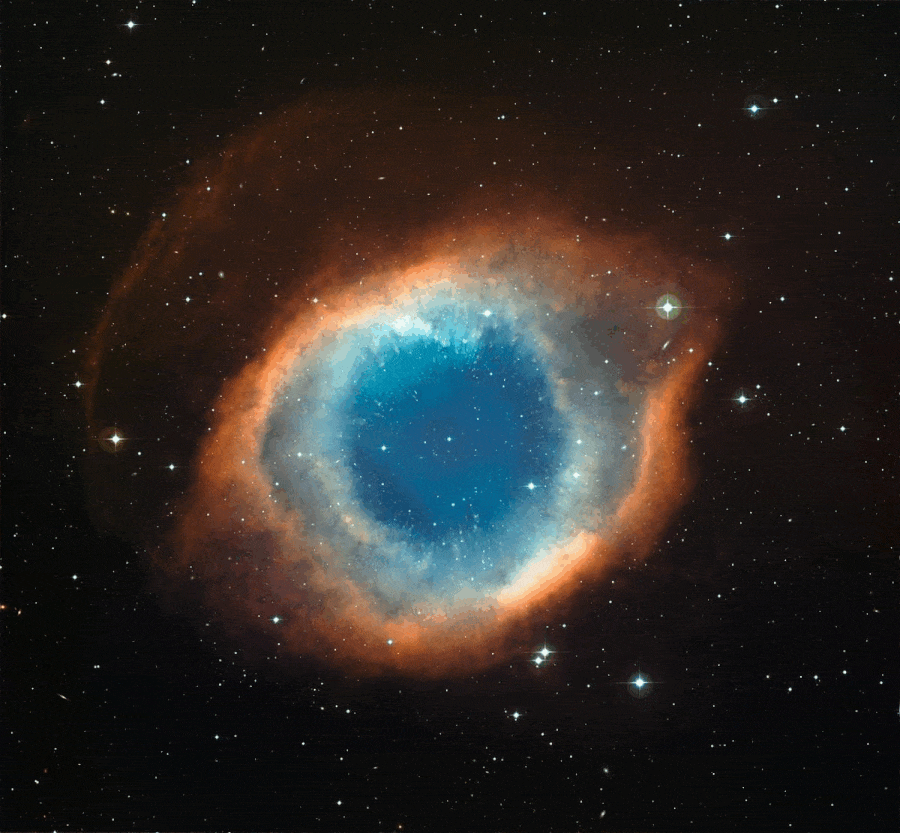
When most of us think of astronomy, we think about our greatest observatories in space: facilities like Hubble and JWST, which continue to break cosmic records and teach us both what our Universe looks like and how it grew up. No doubt, our space telescopes play a major role in bringing new scientific knowledge to the world: one that cannot be underestimated. But no matter how advanced our arsenal of space telescopes becomes, there are still a number of tremendous advantages that we gain from conducting science from ground-based facilities as well. In particular, ground-based facilities have five general advances over space-based ones, and they are:
- Upgradeability. Any new science instrument, set of mirror coatings, or adaptive optics systems can be built and installed on a ground-based facility at any time, with minimal cost and effort. Want to do the same thing in space? It’s one of the most difficult and expensive tasks of all.
- Size. In space, your observatory’s size is limited by the dimensions and the payload capacity of the launch vehicle. On the ground, facilities can be as large and as heavy as we dare to build them.
- Reliability. In the event of a launch failure, a space-based mission is a total loss. NASA’s Orbiting Carbon Observatory is one recent casualty: crashing into the ocean 17 minutes after takeoff, necessitating a total rebuild and relaunch. The risk of such a failure for a ground-based mission is zero.
- Versatility. A network of ground-based telescopes can give you a series of locations to observe even once-in-a-lifetime events. Want to see Jupiter occulting a quasar? An asteroid occulting a background star? The transitional moment of a hybrid eclipse? The chance to capture a Kuiper belt object’s atmosphere? Ground-based facilities give the versatility to capture these events. From space? You rely solely on luck.
- Maintenance. If a component fails, wears out, degrades, or needs an upgrade, what do you do? On the ground, anything can be repaired or replaced, with maintenance facilities often existing on-site. From space? A dedicated (and risky) servicing mission is required.
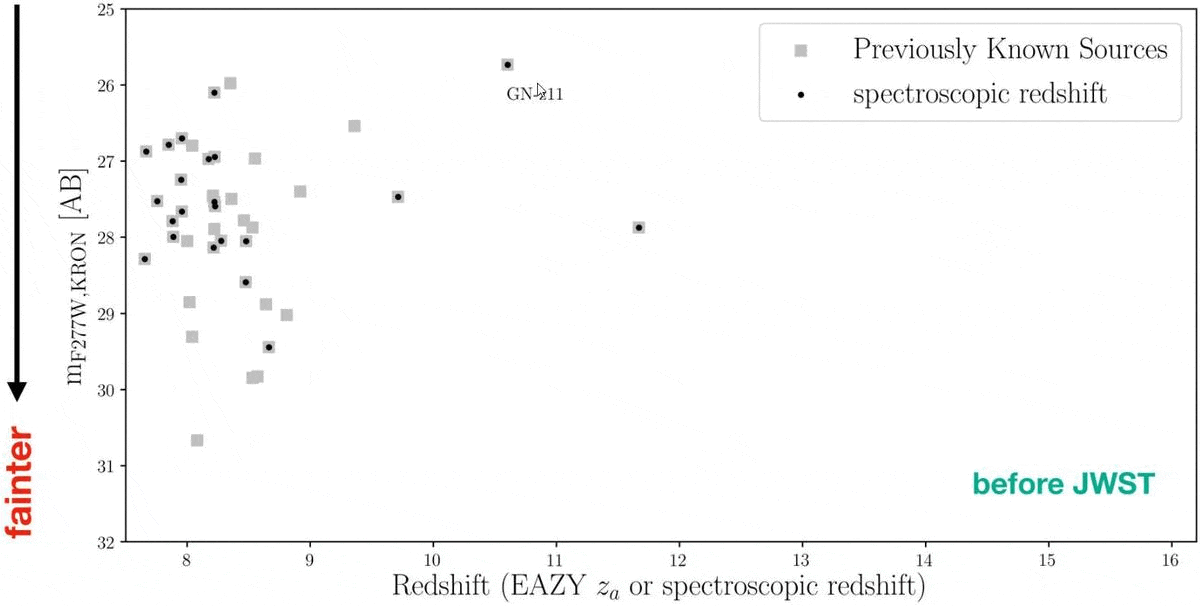
In fact, ground-based facilities play another important role: they supplement space-based observatories by taking some of the pressure off of them. Currently, we can identify an ultra-distant galaxy candidate simply by measuring its light, from space, with an observatory like JWST. In fact, in the very first science image released by JWST, a total of 87 ultra-distant galaxy candidates were found: objects whose light could have been emitted from when the Universe was between 180 and 480 million years old. Yet a full 2.5 years after those candidates were identified, only twelve objects from such early times have been confirmed: with none of them coming from the originally identified field of galaxy cluster SMACS 0723.
Why is that the case?
Because there’s only one JWST up there, and there are thousands of scientifically worthy proposals that seek to use it. At present, only JWST has the capabilities to make those key spectroscopic measurements to definitively tell us whether those “candidates” really are ultra-distant galaxies, or whether they’re less distant, interloping impostors. Those observations are expensive in terms of observing time, meaning that each time we “test” such a galaxy candidate with JWST, that’s another researcher’s novel science program that simply doesn’t get conducted. But if we had a superior ground-based facility — a 30-meter class ground based telescope, in this case — we could perform those spectroscopic confirmation observations from the ground, freeing up JWST to conduct the novel forms of science that only it is capable of.

The way that cutting-edge ground-based science facilities work in this country are relatively straightforward:
- the National Academies of Science, Engineering, and Medicine conduct a decadal review,
- where they issue their recommendations to various agencies (NASA, the National Science Foundation, the Department of Energy, etc.) for funding priorities,
- and then the National Science Board signs off on it,
- so that the NSF director can ask congress for the necessary funding to build the facilities for the new decade (and beyond) of science,
- where after authorization, the Major Research Equipment and Facilities Construction (MREFC) account receives the money for building those facilities,
- and then, once construction is complete, the appropriate division (astronomy, in this case) continues to pay for operations and maintenance costs of those facilities.
This was how a great many of the cutting-edge 21st century facilities were constructed within astronomy and astrophysics. This path, among other endeavors, has brought us our greatest solar telescope of all-time (the Daniel K. Inouye Solar Telescope), the LIGO gravitational-wave observatory that led to the first direct detection of gravitational waves (and more than 100 similar events thereafter), the NSF component of ALMA, presently humanity’s greatest, most powerful array of radio telescopes of all-time, and the Vera Rubin Observatory, which is now completed and is slated to begin science operations later in 2025. All of these endeavors were initially approved and designated as “highest priority” endeavors in the decadal surveys of 2000 and 2010, respectively, and have either recently or are only now coming to fruition.
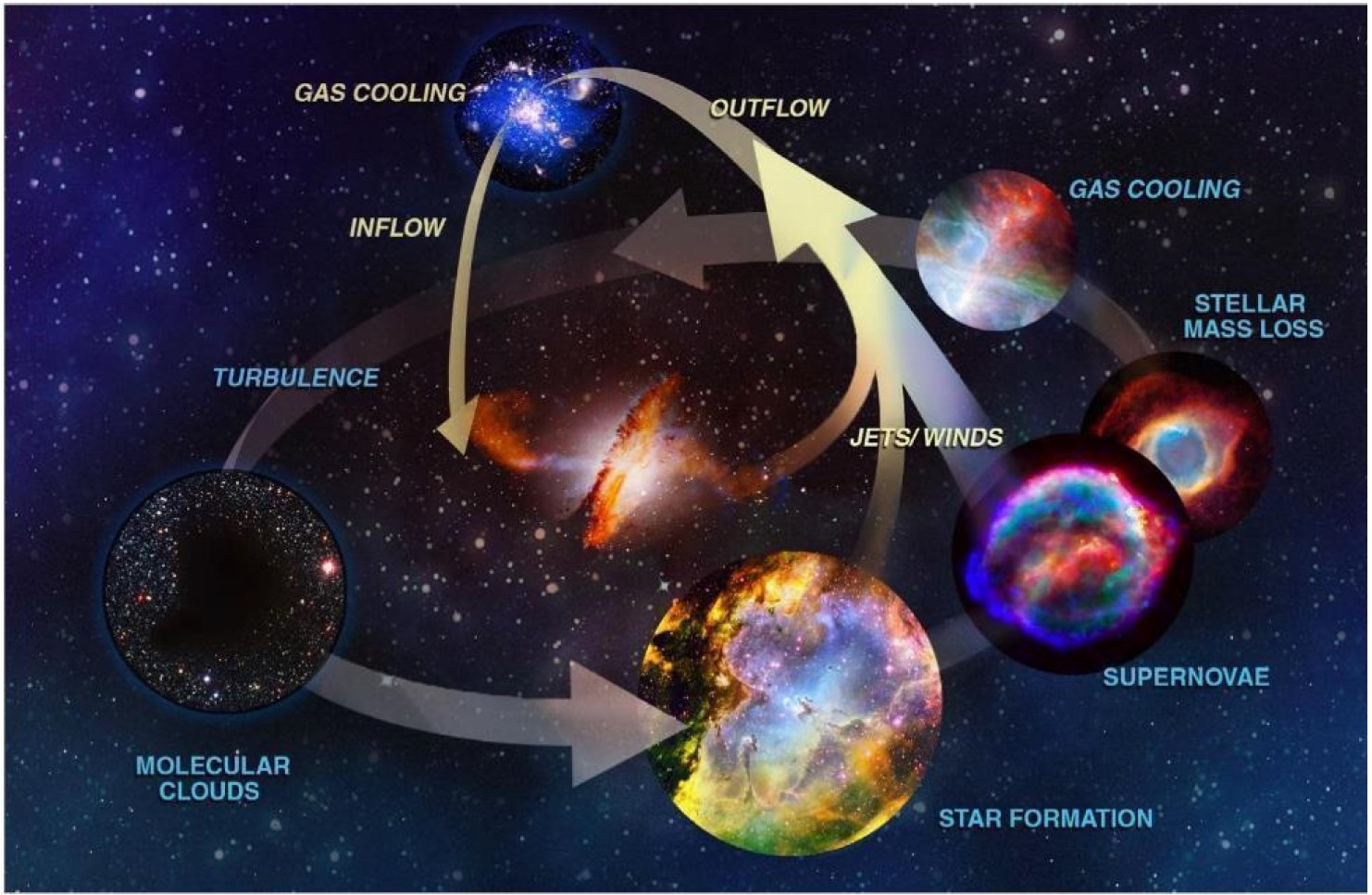
When it comes to the decadal survey of 2020, there were four major “highest priority” endeavors that were selected as ground-based endeavors for the field of astronomy: the Giant Magellan Telescope (GMT), the Thirty Meter Telescope (TMT), the next-generation Very Large Array (ngVLA), and LIGO II: the next-generation ground-based gravitational wave observatory, designed to surpass all of the current generation’s capabilities. Despite broad support across the board for these new facilities:
- within the astronomy and astrophysics community,
- both nationally and internationally, including scientifically and politically,
- within the National Science Foundation and among university and industry partners,
there’s been an obstacle that’s prevented the NSF, uniquely, from acting on these priorities and fulfilling their obligations to the scientific community: the NSF director, who was nominated in 2019 and confirmed in 2020, has neglected to ask congress to authorize the funding to build these new cutting-edge facilities.
That is the one key step — a mandatory step that must occur — that’s preventing the United States of America from building the new cutting-edge facilities that will allow it to remain on the frontiers of science. In the areas of optical/near-infrared astronomy, radio astronomy, and gravitational wave astronomy, in particular, we’re in a “build it to remain competitive at the top, or watch as the rest of the world surpasses us” situation. Here’s a rundown of the facilities we’ll build, but only if we invest in keeping America great.
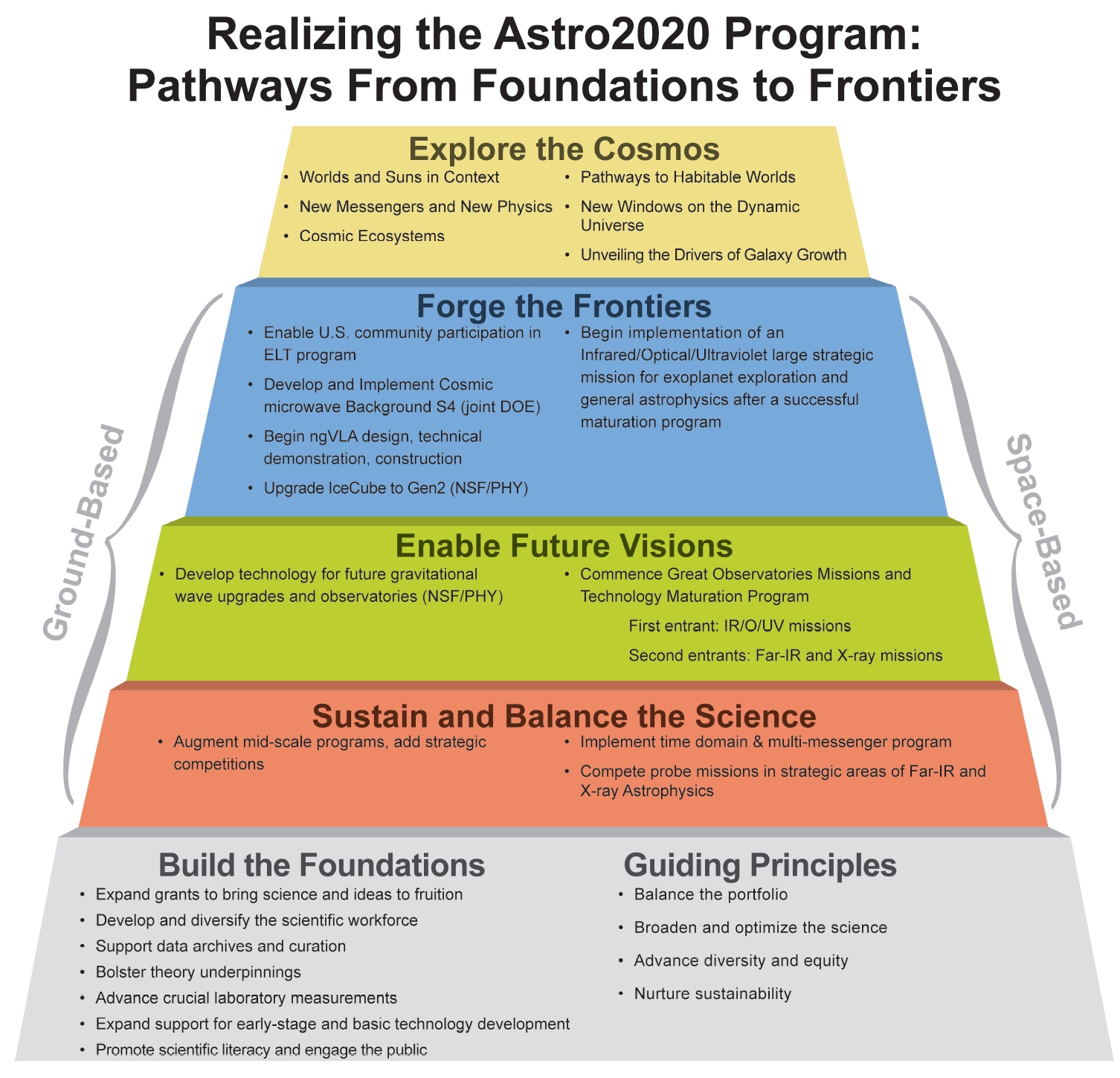
The Giant Magellan Telescope
One of two planned Extremely Large Telescope projects that’s slated to be built and managed by the USA, the GMT is the smallest of the three planned “thirty meter” class ground-based telescopes. However, it’s far from the least powerful, as it contains a number of unique capabilities that make it a superior machine to the other two (the US-based Thirty Meter Telescope, or TMT, below, and the European-based Extremely Large Telescope, or ELT) on a number of metrics. It:
- has the fewest mirror segments, which minimizes one a source of error,
- has a unique design that eliminates diffraction spikes, unique among 30-m class telescopes,
- only has two light-losing reflections, as opposed to five for the ELT,
- is the most upgradeable, as its instruments are the least expensive to build and upgrade,
- and can equal a larger telescope’s performance for a number of science goals simply by observing at a shorter wavelength, as it’s the number of wavelengths that fit across the primary mirror’s diameter that determine resolution.
Currently, the US controls most of the 8-to-10 meter optical telescopes on Earth, but the dawn of the 30-meter era is slated to arrive in just a few years: in the 2030s at the latest. The European ELT is going to arrive irrespective of what the United States chooses to do as far as funding goes, and will indeed become the world’s largest telescope. If the United States wants to remain competitive, we need not only the Giant Magellan Telescope, which will be built in the southern hemisphere, but a 30-meter class telescope that will be in the northern hemisphere as well.
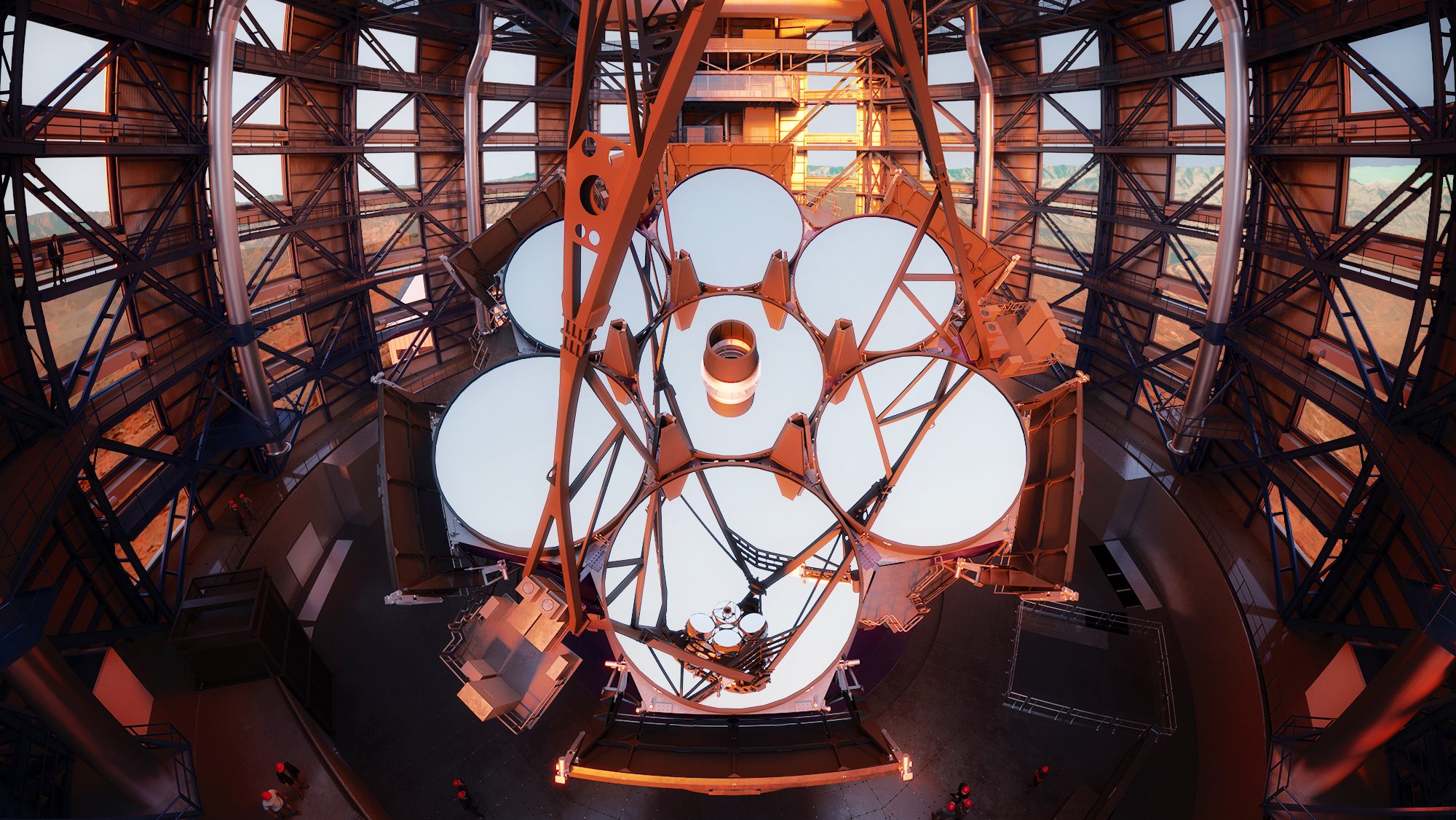
The Thirty Meter Telescope
That’s where the Thirty Meter Telescope (TMT) comes in. Currently, the ELT and the GMT are under construction in the southern hemisphere: both located in the Chilean Andes mountain range. However, there are a great many objects that cannot be observed from the southern hemisphere, and that’s why a northern hemisphere 30-meter class telescope is planned as well. Designed with a very different set of optics and instruments than the GMT, these two facilities are designed to be complementary: where their capabilities overlap, but where each observatory has its own unique aspects of astronomy that it excels at.
From 2019-2022, I was pessimistic that the ideal site for the TMT — the summit of Mauna Kea — would be feasible. However, recent developments have shown the possibility for a path forward for a long-term partnership between astronomers and the Hawaiian community. A new stewardship and oversight authority has been formed, paving the way for astronomy and native interests to coexist, to the benefit of all. There is no more talk about a new construction site for the TMT; talks are about removing several of the older facilities and using a decommissioned site to build the TMT on. There will be more news ahead on this front, to be sure, but a mid-2030s date for completed construction for the TMT, on the desired site of Mauna Kea, remains within reach, but only if the telescope’s construction is selected and funded by the NSF.
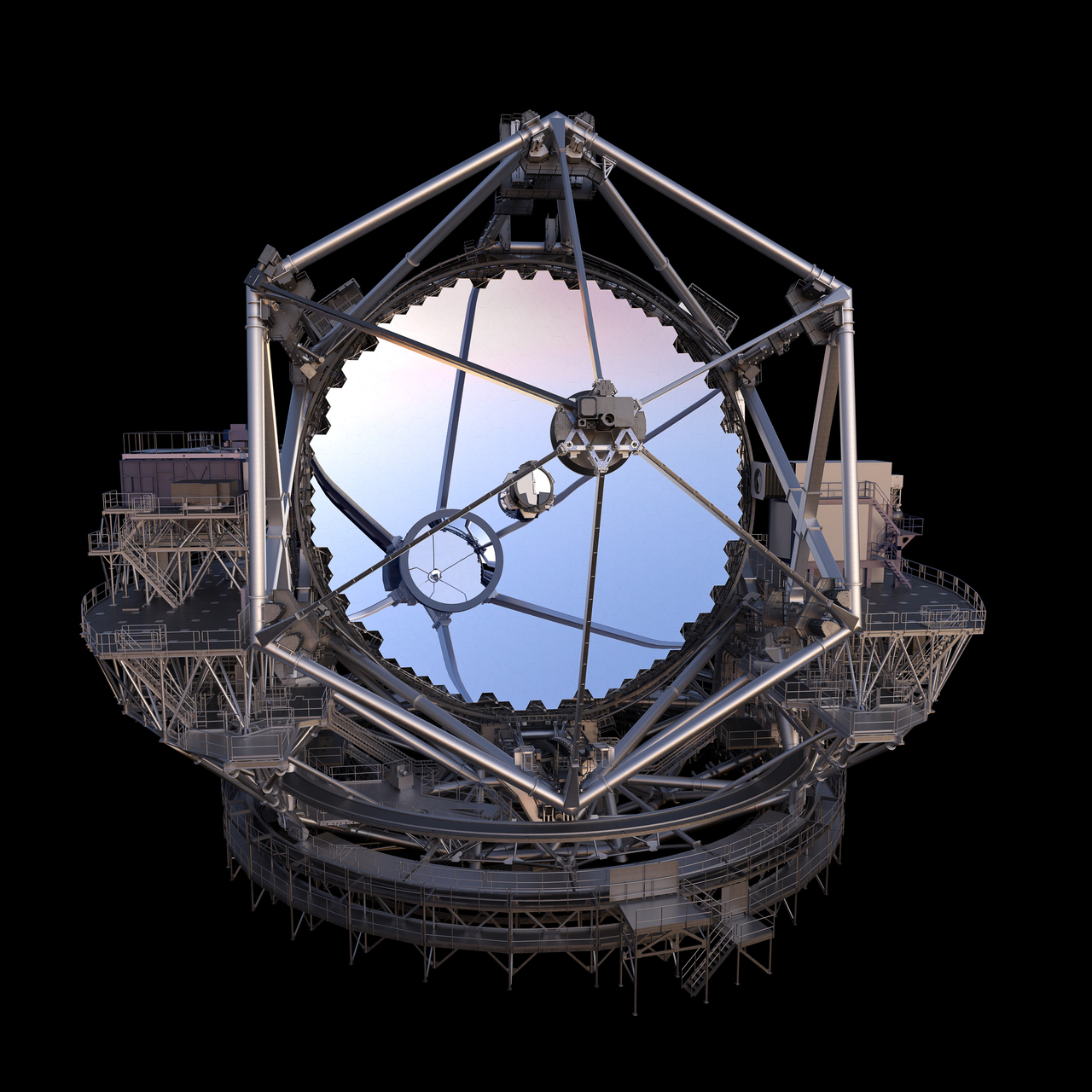
The next-generation Very Large Array
For decades, the United States has been a leader in not just optical astronomy, but in radio astronomy as well. We participate in the Event Horizon Telescope collaboration, which constructed the first images of a black hole’s event horizon. We monitor pulsars using arrays of radio telescopes, where we’ve recently revealed hints of a cosmic gravitational wave background permeating all of space. We identify and track fast radio bursts: a phenomenon whose origin is still being uncovered. And radio astronomy remains one of SETI’s most powerful tools, giving us our best chance of finding intelligent life, beyond Earth, within our own galaxy.
However, Arecibo has collapsed, and the Very Large Array is several decades old. In order to keep up with the rest of the world — which has radio telescope arrays like ALMA, MeerKAT, and dishes that are even larger than Arecibo such as China’s FAST — a new facility is required. The ngVLA, selected as a “highest priority” mission by the Astro2020 decadal, is the preferred option for how to move forward. But unless the money for a new facility comes in, we’ll be relying on old technology, ceding progress in:
- direct imaging of radio sources,
- searching for intelligent signals,
- and monitoring pulsars for gravitational wave signals,
to other nations that have built and maintained these next-generation facilities. The choice is up to us.

Credit: Alex Savello/NRAO
And LIGO II
Back in 2015, advanced LIGO turned on for the first time: poised to monitor the sky in a fundamentally new way: searching for gravitational waves. Almost immediately, as in within just weeks, the first robust gravitational wave signal arrived: GW150914. Fast forward another ten years, and we’ve seen:
- merging black holes,
- neutron star-black hole mergers,
- and neutron star-neutron star mergers, including one with an electromagnetic counterpart,
for a total of over 100 directly detected gravitational wave events. The twin LIGO detectors aren’t alone anymore, either, as they’ve been joined by Virgo (in Europe), LIGO India, and KAGRA (in Japan), with new larger, more sensitive ground-based gravitational wave interferometers planned across the globe.
Will the United States continue to lead the way? LIGO currently outclasses those other facilities, but they’re close to catching up. A new set of interferometer arms, with quieter noise-reducing technology and longer baselines, should allow us to reveal new types of gravitational wave sources and better understand what makes the very fabric of space in our Universe ripple. We have the technological capability and the scientific expertise, and all of these new facilities could be built in the next few years with only a very modest increase in the total NSF budget: from about $9 billion annually to around $11 billion, an increase that’s been long overdue.
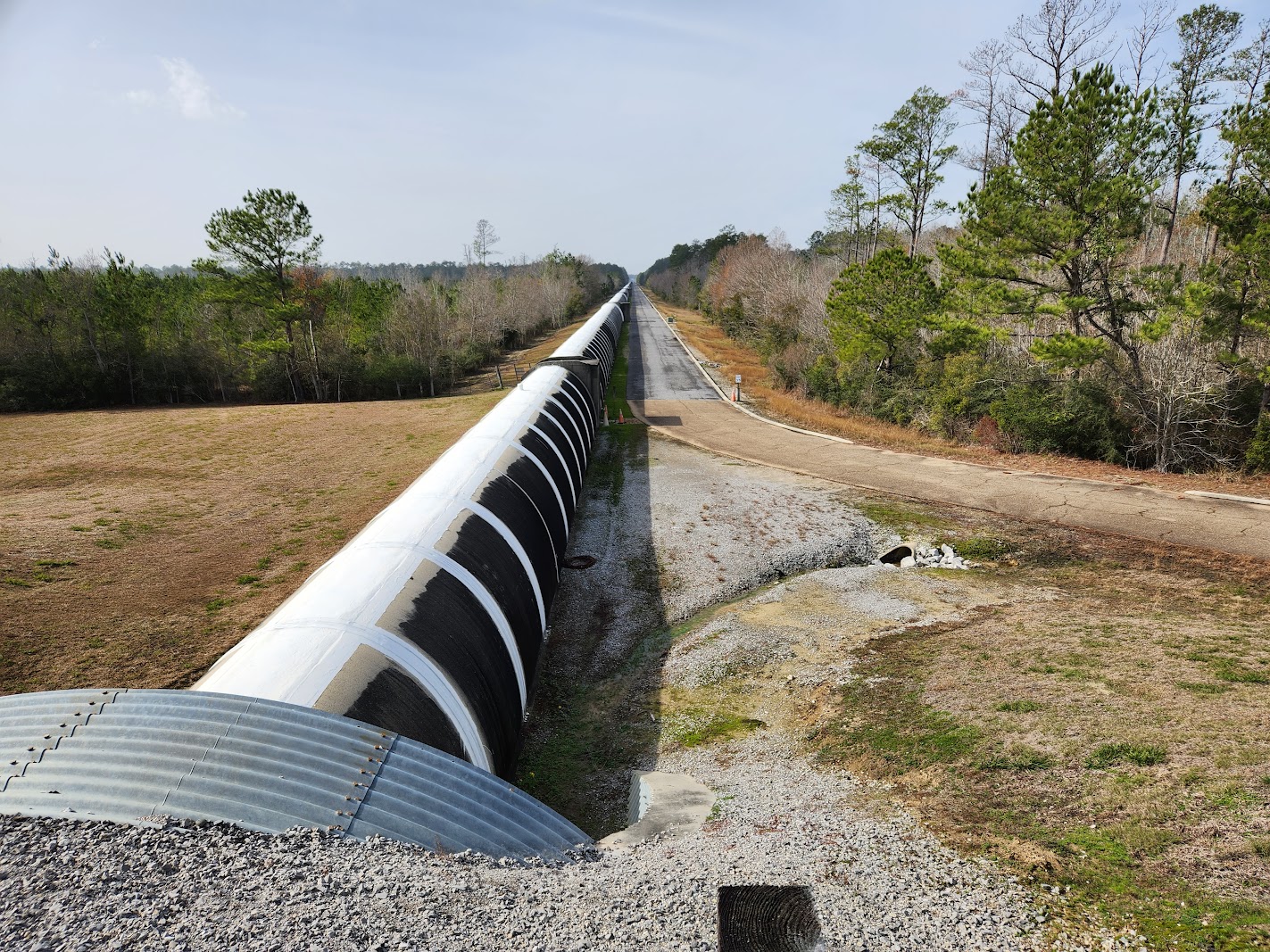
However, if we’re penny-wise and pound-foolish at this point in time, it will be other nations and scientists in other countries that get to answer the current slate of big questions in astronomy and astrophysics. It will be other nations that build the facilities that get to discover those serendipitous phenomena that only appear when a tremendous leap forward in capabilities comes onto the scene. The goal of the NSF, and of the Major Research Equipment and Facilities Construction (MREFC) in particular, is stated very directly: to keep and maintain US leadership in science and engineering. From the MREFC directly:
“Modern and effective research infrastructure is critical to maintaining U.S. international leadership in science and engineering. The future success of entire fields of research depends upon access to new generations of powerful research tools. Over time, these tools are becoming larger and more technically complex and often have significant information technology or cyberinfrastructure components. To be considered for MREFC funding, NSF requires that a major multi-user research facility (major facility) project represent an exceptional opportunity to enable research and education. The project should be transformative in nature, with the potential to shift the paradigm in scientific understanding.”
The time is, at last, at hand to keep America great. If that’s truly our goal, collectively, the time to approve and build these new facilities is now.