JWST fully solves the mystery of “Little Red Dots”
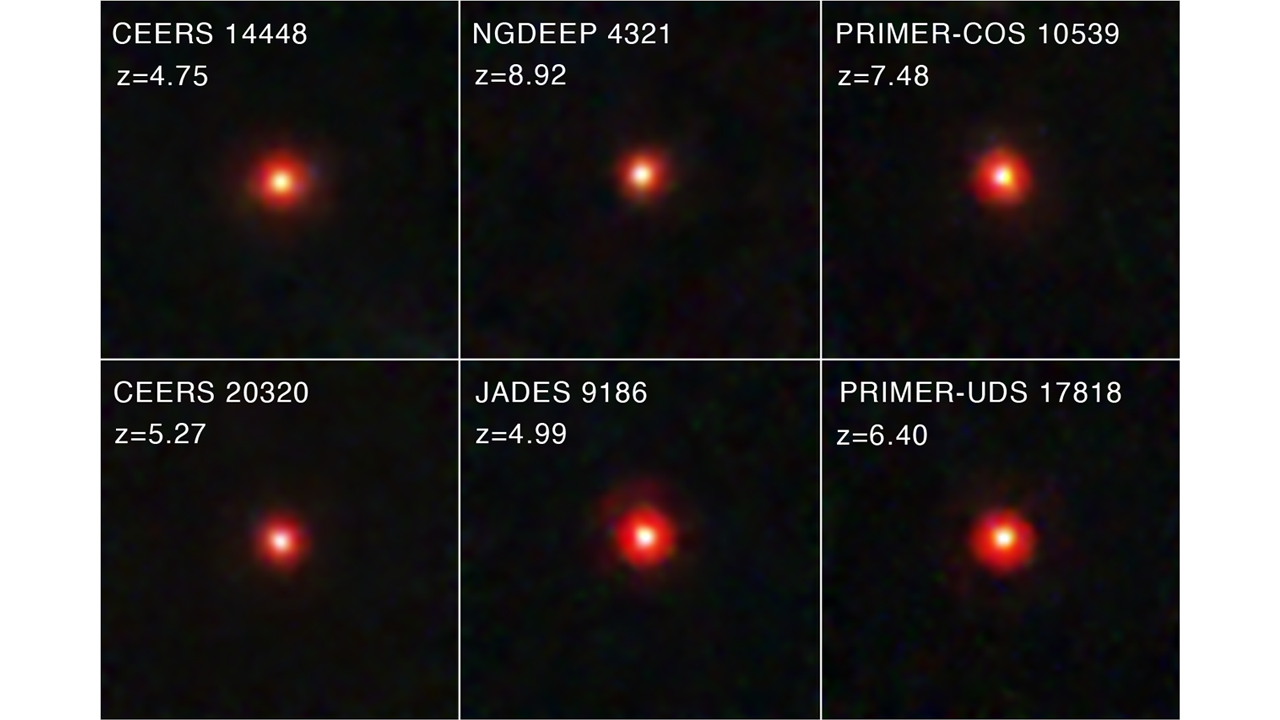
- When JWST first opened its eyes on the deep Universe, the most surprising feature was the large number of bright, red, ultra-distant objects found all throughout space.
- Initially, many astronomers claimed that the abundance and brightness of these objects “broke the Universe,” implying heavier masses and greater star-formation rates too quickly in the early Universe.
- But our understanding of where the light came from within these objects — known as Little Red Dots — was incomplete. Now that we know the answer, things add up once again.
In the summer of 2022, our views of the distant Universe — and our story of how galaxies formed and grew up — changed forever as the James Webb Space Telescope (JWST) began science operations. With superior light-gathering power, it could detect fainter objects than ever before. With superior resolution, it could differentiate single-source objects from extended or multi-source objects better than ever before. And with its infrared-specialized capabilities, it could detect more distant, high-redshift objects than ever before. With the incredible technology aboard JWST, we were poised to learn about the earliest stages of cosmic history as far as stars, galaxies, and supermassive black holes were concerned.
Based on all we had learned previously, we had expectations for:
- how many galaxies we would see early on in cosmic history,
- what the masses and brightnesses of those galaxies would be,
- and how much of that generated light would come from stars, versus how much came from active supermassive black holes within them.
Unexpectedly, a new class of object — Little Red Dots (LRDs) — showed up in great abundance in the data. These LRDs didn’t line up with our expectations at all: they were very bright, which suggested tremendous stellar masses, but were so great in number, at such early times, that the complete picture didn’t add up. Now, at the start of 2025, we’ve finally solved the puzzle, with active supermassive black holes playing an all-important role in explaining the Universe.
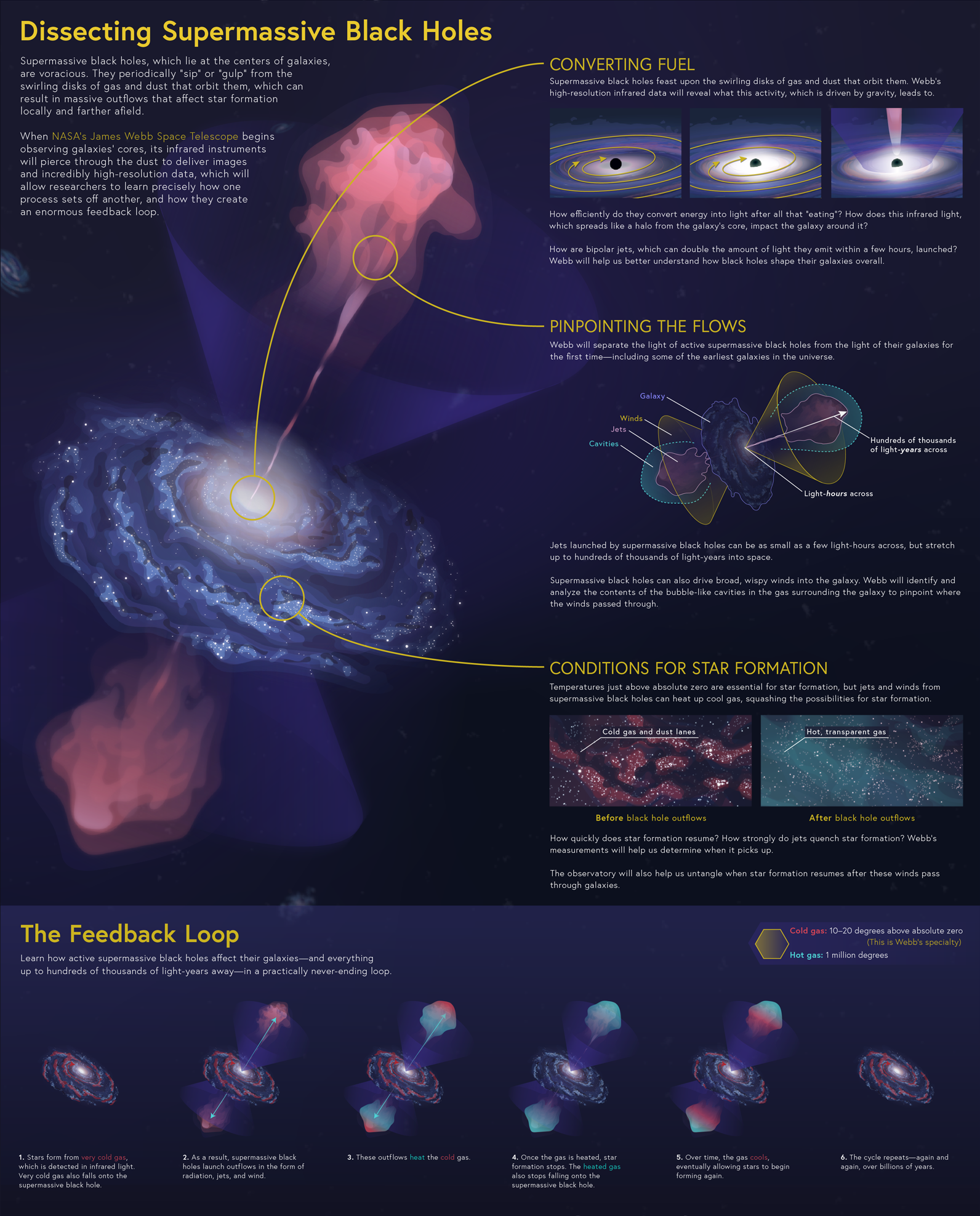
In most galaxies that we see and measure, there are only two major reasons behind why a galaxy would be intrinsically red in color when we observe it.
- The galaxy could be red because the stars within it are primarily old, because the brightest, hottest, bluest stars are also the shortest lived stars. These “red-and-dead” galaxies are mostly gas-free, and haven’t formed any new stars in billions of years.
- The galaxy could be red because it’s very dusty, as cosmic dust blocks the light coming from objects (like stars) that are found behind the dust. This light-blocking dust, because it’s made of dust grains of a specific finite size, is much more efficient at blocking blue light than red light, so this “reddening” effect would apply to all the light produced by the galaxy before it travels throughout the Universe.
For nearby galaxies, we regularly find galaxies that fall into both categories: galaxies that are intrinsically red because they’re old, and galaxies that are intrinsically red because they’re dusty. But early on in cosmic history — just a few hundred million years or maybe a billion years after the Big Bang — there’s no way to have “red galaxies” of that first type. The Universe was simply too young for the hot, blue, luminous stars within them to have all died away.
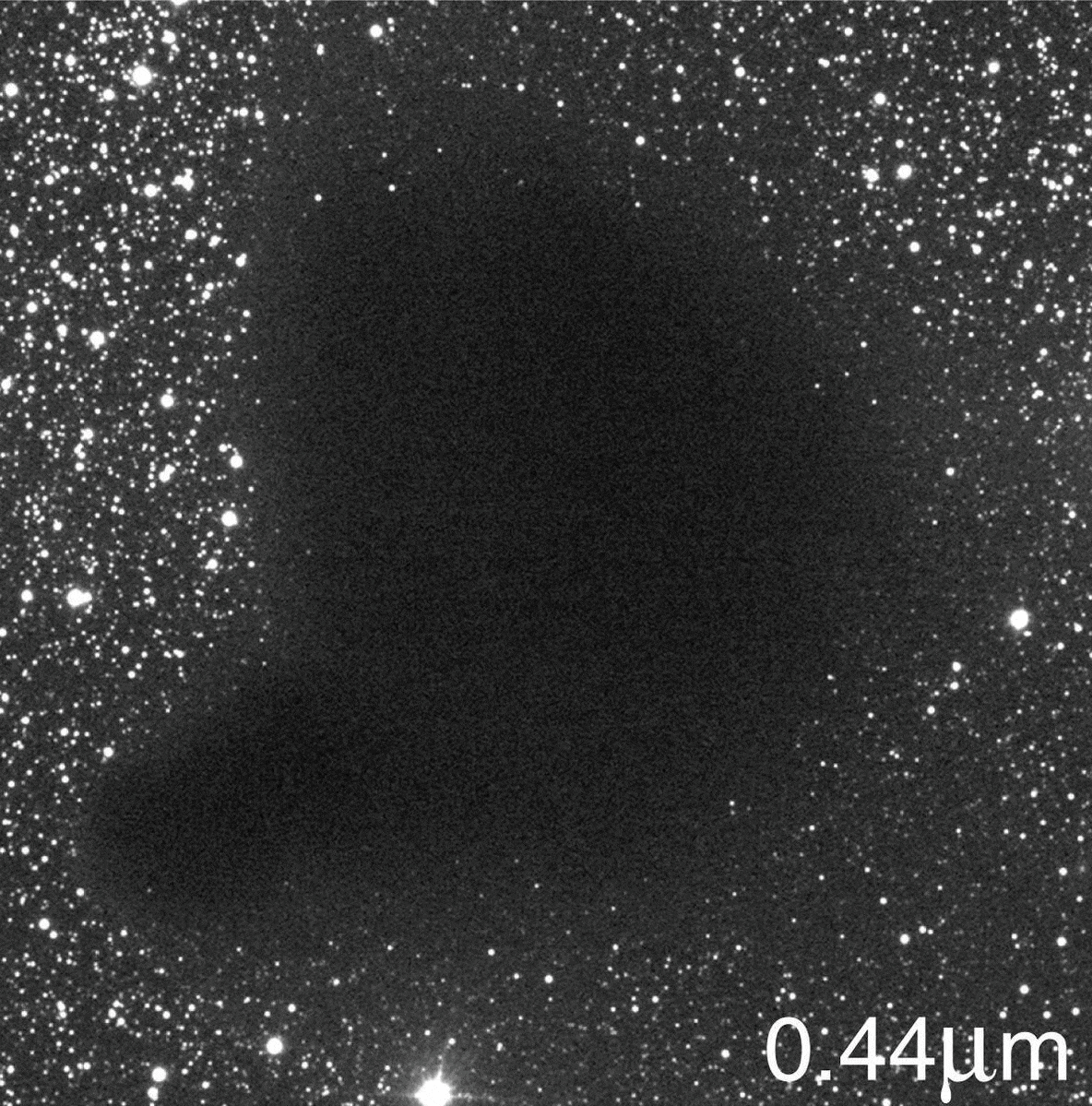
When we see galaxies that are far away, particularly on cosmological scales, there’s another “reddening” effect we have to account for: the effect of cosmological redshift. The farther away an object is from us, the greater the amount of time that the light must travel through the Universe in order to reach our eyes. Because the Universe is expanding, as light travels through it, the wavelength of that light gets stretched — or redshifted — to longer and longer wavelengths. That might make a distant galaxy appear red compared to an otherwise identical galaxy that appears nearby, but we can quantify exactly how.
Relative to a nearby galaxy, the light from a distant galaxy will be stretched, but if we apply a “stretch factor” to the light that we see, we can understand that all wavelengths are stretched by the same multiplicative factor. If you divide the light’s wavelength from the distant galaxy by that “stretch factor” — which is just (1 + z), where z is the redshift of the object — you recover precisely how that galaxy would appear if it were located nearby. You then know that “red and dead” isn’t an option, so you’d naturally chalk up any remaining “redness” that’s observed in that galaxy’s spectrum to dustiness, as dust blocks blue light, but allows red light through far more readily.
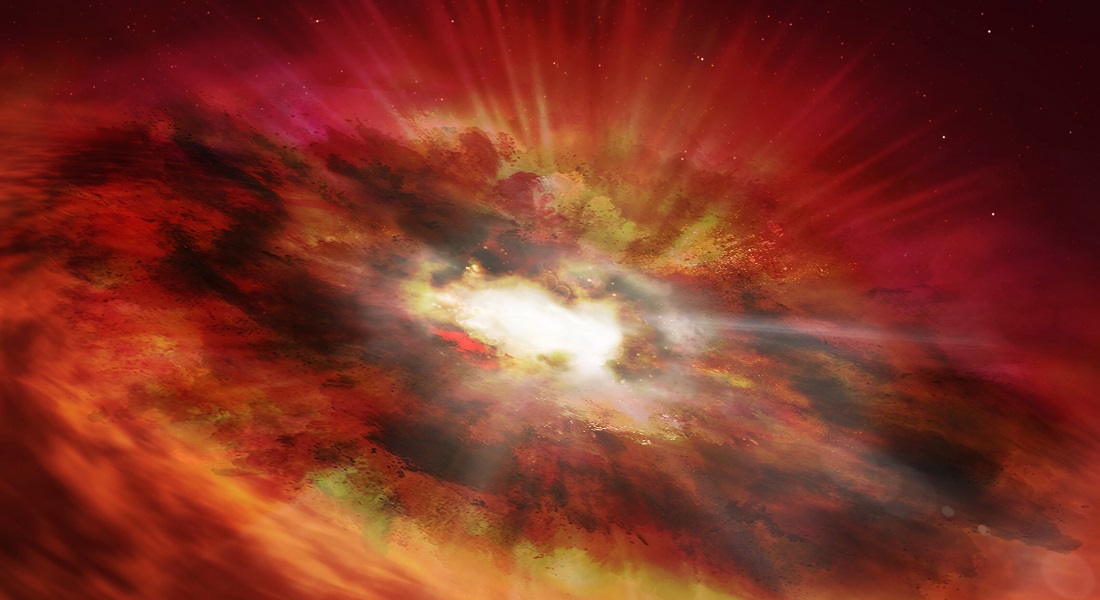
In other words, the simple story is that:
- stars make light,
- dust blocks some of the light (more of the shorter-wavelength, bluer light and less of the longer-wavelength, redder light),
- and then that light travels through the Universe, with all wavelengths of light redshifting by the same “stretch factor” until we observe them.
This simple story worked very well for most galaxies, with only active galaxies — or galaxies with actively feeding supermassive black holes at their centers — such as AGNs or quasars, having a substantial “extra” source of light in addition to stars.
And with all of that in mind, we’re ready to look at what JWST initially saw when it opened its eyes on the distant Universe. In addition to better, more detailed views of objects that we already knew were out there, it discovered an enormous number of objects who were too far away — i.e., whose light was too long-wavelength, due to cosmological redshift — to have been identified and studied before JWST’s unique infrared capabilities. Some of those objects were in-line with what we expected: faint and intrinsically blue, full of young populations of stars that were newly-forming.
But the brightest objects we found at great cosmic distances were these Little Red Dot galaxies (LRDs), and they didn’t line up with our expectations at all.

These LRDs were unlike any other class of object we had ever seen before. When you look at the light they produce — i.e., when you divide out the effects of redshift — you find that the light’s spectrum is flat in the ultraviolet, but then rises at long wavelengths in the optical and into the infrared. The spectrum of these galaxies contain broad emission lines, indicating some type of energetic source capable of exciting enormous numbers of atoms. They’re also tremendously abundant: representing 20% of all broad emission-line sources in the Universe found when the Universe was just 1.2 billion years old or less.
That’s why, initially, these objects were alleged to potentially “break the Universe” as we know it. If the following things were true:
- stars create light,
- dust blocks some of that light,
- that light travels through the Universe,
- and gets redshifted as it travels,
then the only way to explain the brightness of these LRDs would be to have extremely large stellar masses within these galaxies. But this is a problem, because it takes time for structures to form and for matter to collapse in the Universe, and more massive objects take longer periods of time to build up. If these LRDs were obeying the assumptions we just put forth, then there were:
- too many objects,
- that were too bright,
- with too many stars,
- and too much mass within them,
for our current picture of the Universe to explain. That’s why there were so many claims, especially in the early days of JWST in 2022 and 2023, that these Little Red Dots had broken the Universe.
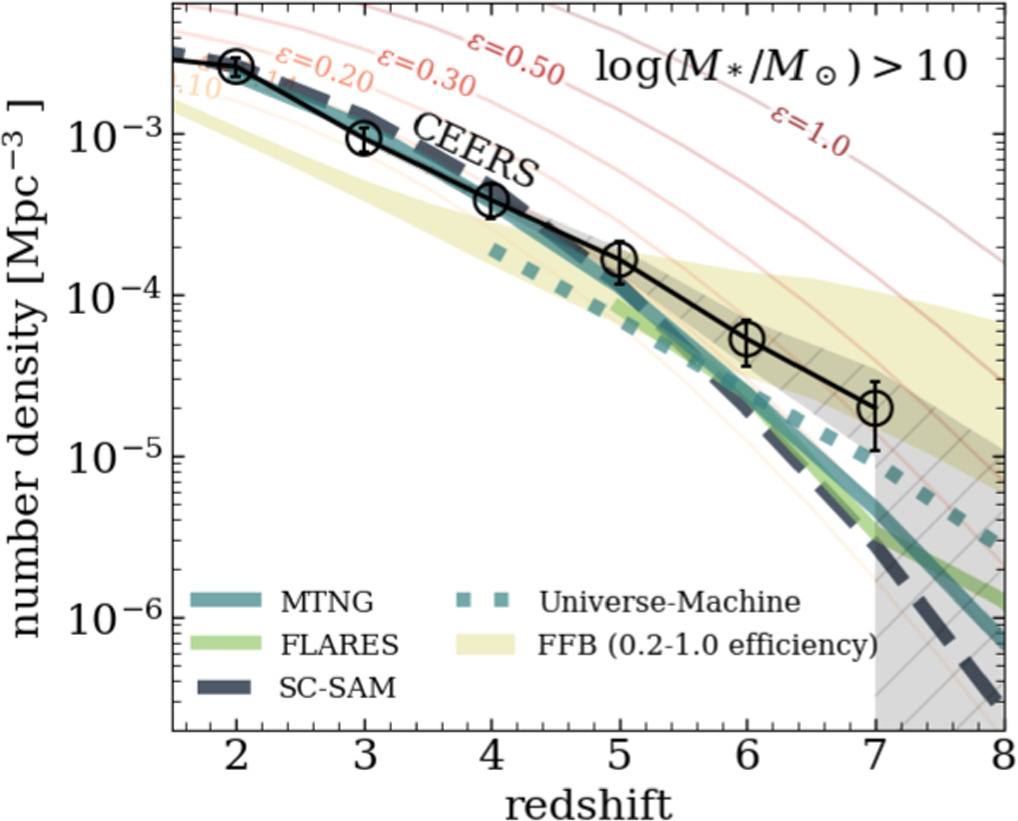
But was the Universe truly broken? Or had we overlooked some aspect of astrophysics in our assumptions about these LRDs?
In science — in general — we must be very skeptical of any observations that conflict with our expectations. We owe it to ourselves, and to the rest of the scientific community, to exhaustively search for potential effects that we have not yet accounted for. One plausible explanation for “enhanced brightness” of these LRDs was that only some of the light came from stars, with the remainder of it originating from an active supermassive black hole at the galaxy’s center: an analogue of a quasar or an AGN.
Many astronomers were quick to reject such a suggestion for a simple reason: we had already observed quasars and AGNs at late-times in the Universe: when the Universe was about 1 billion years or older. We knew how many there were at various points in cosmic time, allowing us to extrapolate what the abundance of quasar-like and AGN-like systems would be even earlier. Yet, when the observations came in, the number density of the LRDs we were finding was about ten times higher than the extrapolations from bright quasars and even more severely over-abundant compared to the known population of AGNs.
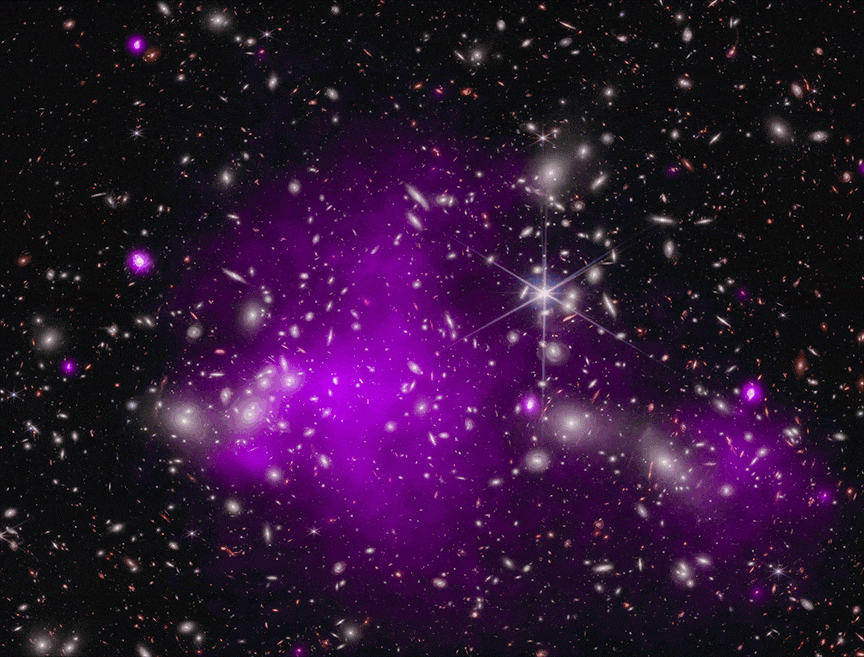
But that didn’t necessarily mean that there weren’t active supermassive black holes contributing to the brightnesses of these LRDs; it could also mean that something breaks down about our naive extrapolations. And indeed — from a completely different line of evidence — there is something that breaks down: the extrapolation that supermassive black holes are only about 0.1% as massive as the total mass of stars within the galaxy that houses the supermassive black hole. As was first shown in late 2023, supermassive black holes can be much more massive, compared to the mass of stars within their galaxies, in the first ~1 billion years of cosmic history than just 0.1% of the total stellar mass.
JWST revealed, particularly in the earliest stages of cosmic history, the existence and great abundance of what we call overmassive black holes: black holes that are significantly more massive than just 0.1% of the overall galaxy’s stellar mass. Many early galaxies have supermassive black holes that are ~1% as massive as the total stellar mass, some early galaxies have supermassive black holes that are ~10% as massive as the total stellar mass, and at least one galaxy has a supermassive black hole that’s just as massive as all the stars within it combined. In other words, compared to the stars within a galaxy, the supermassive black hole can be much more important for the overall brightness of an object than it is even in quasars or AGNs seen in more recent times.

So it’s possible that these LRDs, or at least some fraction of these LRDs, aren’t getting all of their light from stars, but that active black holes are enhancing their brightness. In doing so, they wind up tricking us — if we stay wedded to our naive assumptions — into thinking that we live in a Universe that doesn’t add up.
That’s an idea that might sound plausible to you, but it also might sound ridiculous to you; how an idea “sounds” isn’t necessarily a good arbiter of whether it’s correct or not. Instead, what you want to do is measure a large sample of the relevant objects — the LRDs, in this case — with sufficient precision so that you can learn about their properties, and determine whether this potential explanation is viable or not.
That’s precisely what a study performed by a large collaboration of scientists working with JWST data, led by Dale Kocevski, just accomplished. They took a census of LRDs from a variety of JWST observing programs, including CEERS, JADES, NGDEEP, PRIMER-UDS, PRIMER-COS, and UNCOVER. From the first 1.1 billion years of cosmic history, they identified 341 LRDs, and revealed an enormous amount of information about them: information that could show whether supermassive black hole activity could bring these objects back in line with our picture of how the Universe grew up.
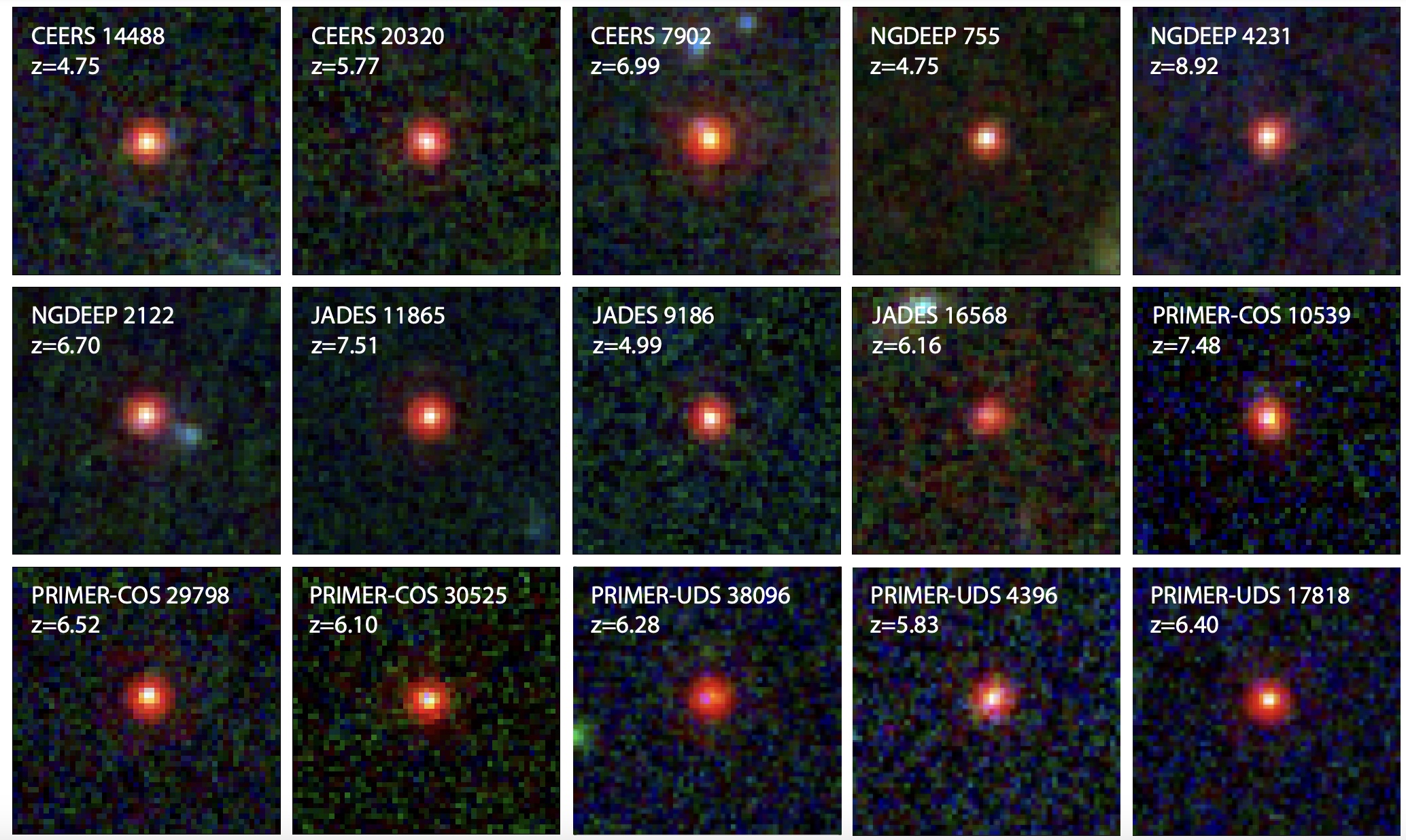
So, what did they learn about these Little Red Dot objects? A ton, including:
- that at least around ~70-80% definitely possess accreting black holes,
- that they practically disappear when you look more than 1.2 billion years after the Big Bang,
- but they peak about 900 million years after the Big Bang,
- and then are less abundant farther back,
- but are found quite long ago, at least as far as about 400 million years after the Big Bang.
The LRDs that do contain evidence for supermassive black hole activity also display some of the strongest evidence for “overmassive” black holes: where the inferred mass of the supermassive black hole is more than 0.1% of the total stellar mass of the galaxy.
However, these LRDs appear to be something unique when compared to other known objects. Do they have dusty toruses surrounding them like known quasars and AGNs do? We don’t know; there’s no observational evidence that indicates that the answer is “yes,” but we can’t rule it out. Of the 341 LRD galaxies singled out so far, only two of them exhibit X-ray emissions: making them the first LRDs known to emit them. And when you look at the light that they generate, the “flat, rest-frame ultraviolet” light must be due to stars within the host galaxy, while the “rising-at-long-wavelengths, rest-frame optical and infrared” light must be due to the contributions from the active supermassive black holes within them.
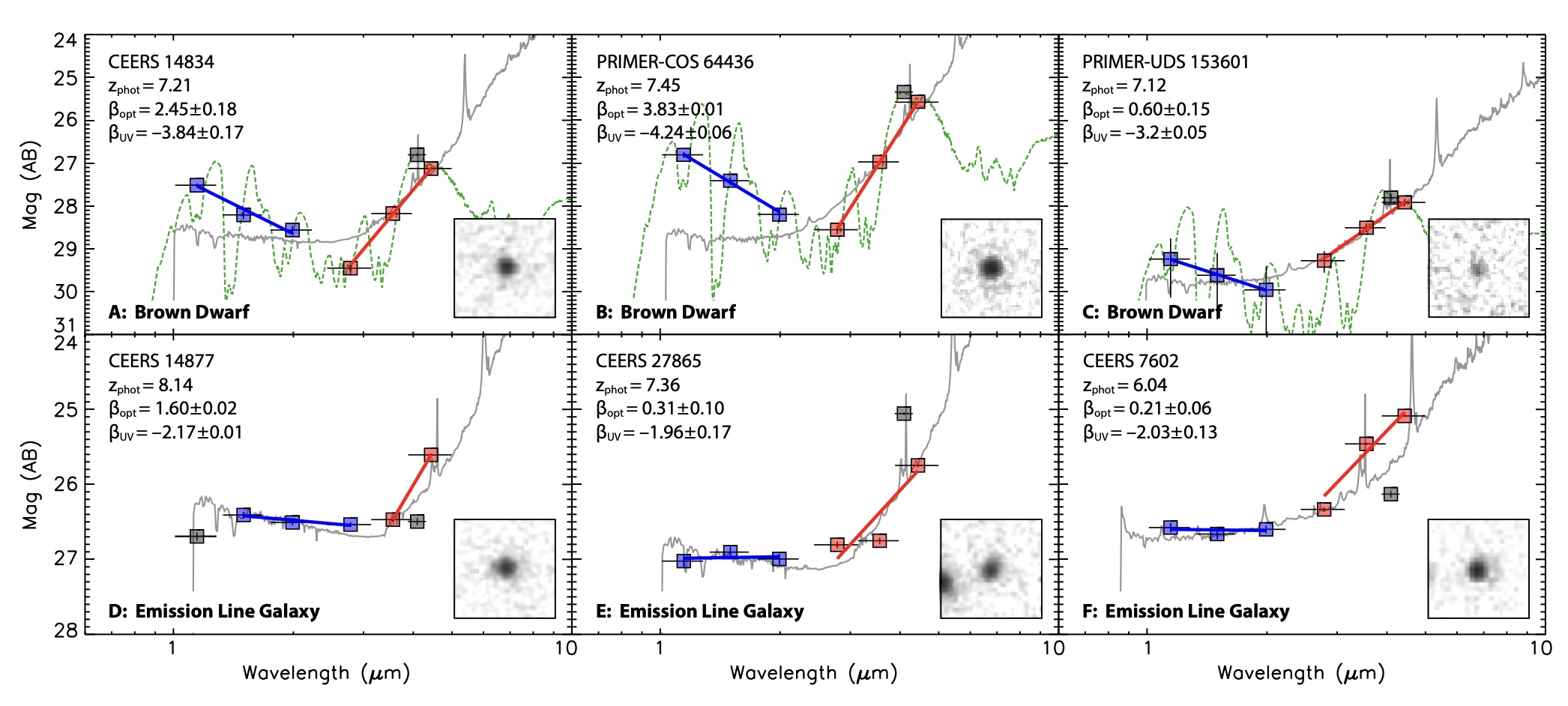
That’s the key piece of the story: the one that allows us to solve the puzzle. We know that these Little Red Dots have contributions from stars within the galaxy and also from the activity of the central black hole. Because you can quantify which components of the light you observe (the flat, rest-frame ultraviolet) is caused by the stars in these LRDs compared to the components (the rising, rest-frame optical and infrared) caused by the active black hole, you can make estimates for:
- the stellar mass of each galaxy,
- the central black hole mass in each galaxy,
and then determine both how massive the galaxy is and just how “overmassive” each black hole is.
It’s only by gathering this data and performing such an analysis that we can draw the ultimate conclusion: about whether these LRDs do in fact “break the Universe,” or whether the now more-accurately-measured properties of these LRDs are consistent with the concordance cosmology used to describe the rest of the Universe. As you might have guessed, cosmology has survived, and the Little Red Dot galaxies we see can be explained by a stellar component plus an active black hole component, rather than by stars-and-dust alone. Now that the Universe is safe, the next steps will be to learn more about the nature of these LRD objects, to investigate their formation, evolution, properties, and the nature of their disappearance as time passes and the Universe grows: from its infancy into cosmic adulthood.